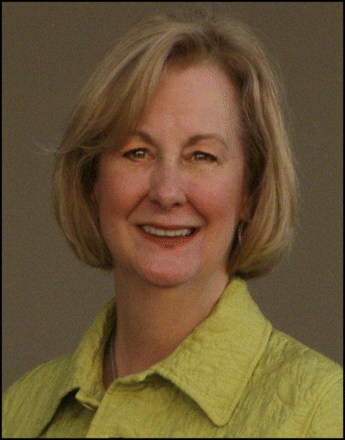
Kathy Hunt, MS, CNMT
Just as biology is divided into systems, organs, and cells, so is the evolution of imaging. In the past, imaging focused mainly on anatomic imaging of systems and organs. The exception was nuclear medicine imaging, which also focused on systems and organs, but the images were based on physiologic principles due to the molecular properties of the radiopharmaceuticals. Being physiologic, nuclear medicine is a natural mesh with current attempts to image down to the smallest level possible––the molecules and even cells that make up the molecules in disease processes.
Our field is the cornerstone of molecular imaging. It was the first imaging modality that was physiologically based. Nuclear medicine began with assessing the function of organs by imaging and quantifying iodine uptake in the thyroid gland. The field expanded as we obtained the ability to assess bone metabolism by evaluating the hydroxyapatite system, and the applications of bone scanning were expanded even further as we developed the instrumentation to acquire 2-dimensional whole-body scans. The field then expanded into cardiology, and as our instrumentation continued to progress to tomography, we were able to assess the coronary blood flow and to render a 3-dimensional image of a beating heart. As we are now expanding into oncology, we are performing whole-body scans to assess glucose metabolism or cell proliferation with positron emitters, such as 18F-FDG and 18F-labeled 3′-deoxy-3′-fluorothymidine (18F-FLT), and have the ability to fuse these images with anatomic images. This is the foundation of molecular imaging, but not all nuclear medicine procedures can be defined as molecular imaging.
The generally accepted definition of molecular imaging is the visualization, characterization, and measurement of biologic processes at the molecular and cellular levels in humans and other living systems. Molecular imaging starts with visualizing biologic processes, such as cell growth or cell destruction, which lead to biochemical changes. We know from our nuclear medicine training that changes in physiology and biochemistry occur before changes in anatomic structure. PET is being used as a molecular imaging agent when 18F-FDG or 18F-FLT is used for the purpose of visualizing cell metabolism and proliferation during the disease process. These studies can assess whether a tumor is responding to a particular chemotherapy agent. Examples of other techniques that rely on visualizing biologic processes are MR spectroscopy, which can identify proteins produced during the disease process, or bioluminescence imaging, which targets gene expression.
Molecular imaging also uses strategies to characterize this biologic activity. For example, these techniques can distinguish rapidly dividing cells from cells in stasis and can depict the formation of new blood vessels, referred to as angiogenesis. Characterization often includes estimating the relative rates of cellular changes and quantifying the changes under observation, leading to measurement of the disease process. This is in contrast to the classic form of diagnostic imaging, which documents findings that show the end effects of the molecular alterations.
Measurement is similar to characterization but has a very quantitative aspect. To measure from molecular imaging techniques requires data extraction from the images, not just interpretation of the images themselves. For example, measuring a standardized uptake value on a PET scan involves documenting the exact time of imaging, the patient's height and weight on the day of the procedure, and the counts from regions of interest. From these measurements, the technologist can calculate a relative concentration of activity in a specific target area. This measurement can also be used to quantify how much the concentration in the target area changes over time. The standardized uptake value is completely dependent on excellent technique by the technologist. For this measurement to be useful in monitoring the disease over time, it has to be quantitative and reproducible.
Currently, nuclear medicine leads the way and plays a huge role in the clinical practice of molecular imaging. Molecular imaging is made up of many imaging techniques, some of which are used in human studies now, such as MR spectroscopy and PET, and some which have not progressed that far, such as optical imaging. Presently, modalities such as PET, functional MRI, MR spectroscopy, and the use of microbubbles in sonography give more physiologic information and even some information at the cellular level. The use of hybrid imaging instrumentation and coregistration also allows us to combine anatomic and functional information into a single acquisition.
So, what does the future hold? As molecular imaging develops, hybrid imaging using nuclear medicine will remain an integral part of molecular imaging. The current emphasis is placed largely on PET imaging with biomarkers. However, single-photon receptor imaging and other nuclear medicine procedures will move into the molecular imaging arena.
Nuclear medicine is not going away––we serve an important role in disease management. But our focus is going to change. Currently, in our daily nuclear medicine work, the primary endpoint is the image itself, which is interpreted in relation to known patterns and appearance of disease. We image physiologic processes, sometimes cellular, but mostly on the system or organ level. Clinical research in nuclear medicine is introducing new radiopharmaceuticals, or biomarkers, and optimizing current procedures. This research typically takes place in human clinical trials, and that is why the SNM Clinical Trials Network (CTN) is important in advancing nuclear medicine and molecular imaging.
The CTN provides a mechanism for rapid translation of developments in cellular and molecular biology into the clinical care of patients through translational research––research that is transferred from the preclinical setting to the clinical setting. The process begins with discovery and development in the laboratory, followed by further assessment at the preclinical level with small-animal studies. If successful, the studies then progress to human clinical trials. The CTN is the link between facilitating the development and assessment of new drugs and innovative therapies and supporting industry in developing novel imaging biomarkers.
Molecular imaging is allowing a more personalized approach in the detection, evaluation, and management of disease. The patients will benefit from earlier, more accurate diagnoses and safer, more effective treatments. Personalized imaging techniques will be able to predict what type of scan or diagnostic study is needed for an individual with a specific disease. Simply stated, we will use the right molecular probe or biomarker for the right target to monitor the right disease in the right patient. We can then predict the best treatment options for an individual. So with respect to therapy, personalized medicine means finding the right drug against the right target to treat the right disease in the right patient. By individualizing treatment plans, it is hoped that 2 things will occur: first, patient outcomes will improve because certain treatments will be eliminated for predicted nonresponders, and second, overall health care costs will decrease as a result of properly utilized resources.
As technologists, we will need to become familiar with the basic pharmacologic aspects of the biomarkers and probes used in molecular imaging. We will need to apply this knowledge when performing the procedures and particularly when imaging to monitor the effects of therapy. These new modalities and techniques will require an increase in education with an emphasis on cellular biology, statistics of data collection, and clinical experience involving other imaging modalities. The emphasis on data extraction will have to be supported by consistency in the way technologists perform studies in order to obtain reproducible and accurate data.
All this will change the way nuclear medicine technologists do their job. First, we will be more intimately involved in clinical trials. Protocols for clinical trials are much different from protocols for general imaging. Strict research procedures and methodologies must be followed. Also, molecular imaging will be the common diagnostic test. We will be imaging as part of a therapeutic protocol. The results of our imaging can lead to a change in the course of therapy and can also be used to predict potential responders, as well as nonresponders. The drugs of the future will be designed on the basis of a disease- or patient-specific molecular signature. This precise level of detail provides critical information not only for diagnosis but also to help determine what therapy will be most effective for any given patient.
In conclusion, molecular imaging with the creation of biomarkers and probes leads to the practice of personalized medicine. This approach will improve diagnostic effectiveness and patient outcomes. Individualized imaging will lead to early detection, and the design of individualized treatment plans will be based on the patient's genetic makeup or the specific biologic processes involved in the disease. When resources are specific for the patient, the overall effect will be decreased expense and better care for the patient. Expensive treatments that are not going to be effective will not be used, and a more effective treatment can be chosen.
Although it is impossible to predict the future, it is extremely likely that molecular imaging will continue to grow. We, as nuclear medicine technologists, need to continue to educate ourselves and be a vital part of that growth.